Generating power from organic waste (palm, crops, manure) and achieve cost saving in long run.
Intellectual Property (IP) Rights:
Title Of Invention Patent Filed : Aerobic Acidophilic Bio-Desulfurization Of Biogas (Hydrogen Sulfide)
Malaysian Patent Application No.: PI 2017703879
International Patent Cooperation Treaty (PCT) Application No.: PCT/MY2018/050068
BIOGAS BASICS
As a Total Solution Provider, UESB are also able to provide attachments for use in Biogas Plant i.e. Biogas Generator Set, Biogas Blowers and Biogas Dryers. We offers Biogas Generator Set for captive power purpose and also export to grid (FiT). With our personnel background from electrical power generation and also wide experience in biogas capture, we are able to deliver package to the requirements of customer and also in line with good industry practices of the biogas sector.
Biogas Blowers are designed for biogas delivery systems where corrosion and leakage prevention are additional factors aside from efficiency and operational safety. The blowers able generate smooth pressure with less overshoot and fewer pressure fluctuations.
We provide Biogas Dryers that are machines for reducing water content in biogas which effectively removes condensate from the system to allow for maximum biogas utilization and increased combustibility means less wasted biogas.
Biogas fermentation, also known as anaerobic digestion, refers to the process of biogas production through decomposition of organic matter by many varieties of fermentation microorganism under anaerobic conditions. Biogas production is a complex biological process. The three-stage theory is commonly accepted, as shown in figure below.
First Stage
The first stage is hydrolysis. In this stage, complex organic matter is decomposed into simpler forms by extracellular enzymes of anaerobic bacteria, such as cellulose’s conversion into simple saccharine, protein’s conversion into aminophenol, and fat’s conversion into fatty acids and glycerin. Then the simple organic matter converts into acetic acid, propionic acid, butyric acid and ethanol through anaerobic fermentation and oxidation by acid-producing bacteria. The dominant fermentation bacteria participating in this process are anaerobic bacteria and anoxic bacteria.
Second Stage
The second stage is the hydrogen-producing acetogenic stage. In this stage, hydrogen-producing acetogenic bacteria convert the intermediate products of the first stage, such as propionic acid, butyric acid and ethanol (but not acetic acid, formic acid and methanol) into acetic acid and hydrogen. This process is accompanied by CO2 generation.
Third Stage
The third stage is methanogenesis. In this stage, methanogenic bacteria convert acetic acid, hydrogen, and CO2 produced in the first and second stages into methane.
CONDITION OF BIOGAS FERMENTATION
The essence of biogas fermentation is a series of microorganism activities. In microbiological terms, biogas fermentation is a process of cultivating anaerobic bacteria that have high activity to obtain high biogas yield.
In biogas fermentation, the raw material is the chief link in the process as the substrate of biogas production. The characteristics of the raw material, such as temperature, pH, carbon-to-nitrogen ratio, nourishment, microelements, toxic matter and others, determine the rate of digestion, the fermentation time, and the rate of biogas production.
All kinds of livestock or animal waste, such as pig, cattle and chicken manure, are good raw materials for biogas fermentation. Furthermore, all kinds of crop residue, agricultural castoffs and wastewater from production of alcohol, starch, palm oil and bean products can be used as raw material for fermentation.
BIOGAS TECHNOLOGY
A typical CSTR biogas plant includes the following major components as shown in figure below.
Pretreatment

No matter what type of biogas technology is selected, pretreatment of substrate is usually the most important precondition to ensure the stable operation of the digester. There are several reasons to pretreat substrates before the fermentation, including:
- Sorting and removal of disturbing/harmful substances
- Buffering and homogenization
- Adjustment of the total solids or CODcr
- Comminution
- Heating or cooling (adjustment of substrate temperature)
- pH adjustment or nutrition balance
- Inoculum or digestate reuse
Digester
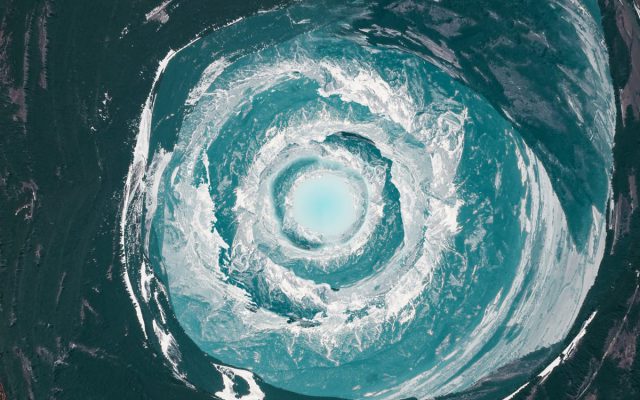
Fermentation is the core technology of a biogas plant. Anaerobic digestion has a variety of classification methods. Continuous stirred tank reactor (CSTR) is one of the most successful and popular types.
CSTR is a reactor with stirring device that keeps the fermentation materials and the microorganisms in the state of complete mixing. The efficiency is obviously much higher than in a conventional digester like a covered lagoon. Thus, CSTRs have been commonly used in mid- to large-scale biogas projects, since far more compact than lagoon-based digesters. Additionally, a properly designed, constructed and installed mid- to large-scale steel tank CSTR system is generally much safer than a covered lagoon system, which is prone to tears and leakage.
Operationally, the substrate is periodically or continuously added to the CSTR digestion tank. After mixing and contact with the anaerobic bacteria in the tank via anaerobic microorganism adsorption, digestion and biodegradation, the organic matter is converted into biogas. The biogas is discharged from the top, while the fermented substrate is discharged from the upper parts of the reactor.
Temperature is an important factor for anaerobic digesters. Temperature affects the microorganism growth rate and substrate metabolism rate by affecting the enzymes inside the anaerobic bacteria. Consequently, temperature affects the sludge yield rate, the removal rate of organic matter, and the loading rate of the anaerobic process.
Anaerobic microorganism can be divided into two major types, as shown in figure below.
Thermophilic Fermentation
The optimum temperature range of thermophilic fermentation is 50-55°C. Thermophilic fermentation has a higher biogas yield rate than mesophilic fermentation and can kill or reduce pathogens and parasites effectively. But it is not easy to use thermophilic fermentation in biogas projects, for two main reasons:
- The energy cost of keeping a thermophilic temperature is higher than for mesophilic digestion. Without proper heat recovery or an inexpensive heat supply, thermophilic digester operation could be costly.
- High heat may be disadvantageous to the digestion reactions if the NH4+-N ratio is high in some substrates. Thermophilic microorganisms normally cannot tolerate the same high level of NH4+-N ratio as mesophilic microorganisms. This means the bacteria acclimation could be an issue for thermophilic processes.
Mesophilic Fermentation
Mesophilic fermentation, the most common fermentation process, has an optimum temperature range of 35-38°C. It is important to note that 45°C is a disadvantageous temperature for any anaerobic process – it belongs to neither the mesophilic scale nor the thermophilic scale, but lies between the two. The activity of anaerobic microorganism will be always low at this temperature, and therefore operation of an anaerobic reactor at this temperature should be avoided.
Anaerobic organisms are sensitive to temperature fluctuation: The bacteria can die when the temperature exceeds the upper limit of the growth temperature range. If the temperature is too high and/or persists for too long, the bacterial activity may not recover, even when the temperature is restored to the normal operating window.
When the temperature exceeds the lower limit of the growth temperature range, the bacteria will not die but will stop working or enter a slow metabolism condition and stay dormant. The lower the temperature becomes and the longer the low-temperature state persists, the more the biogas production rate is reduced and the more difficult it is to recover when the temperature is returned to normal.
It is critical to point out that the temperature sensitivity of anaerobic digestion performance is directly related the organic loading rate. Therefore, it is important to maintain stable a reactor temperature when the organic loading rate is high. Temperature fluctuation should be controlled within ±2°C per day.
In order to ensure digestion efficiency and biogas output, mesophilic or thermophilic fermentation is commonly used. The heating device is set outside the reactor or on the reactor walls to maintain the fermentation temperature.
CSTR (as shown in figure below) is also called high-speed biogas fermentation. Properly designed CSTR systems can operate at high solid concentrations (8-12% total solids). The advantages of CSTR are large digestion capacity, large-quantity biogas production, convenient operation, easy start-up, and low operation costs. Generally speaking, a CSTR reactor is applicable to all kinds of substrate.

Desulphurization
Biogas produced through anaerobic digestion mainly contains CH4 and CO2, together with some trace gases, such as H2, N2 and H2S. Usually, the percentage of CH4 is 55-65%, and that of CO2 is 35-40%. Attention must be paid to H2S in the biogas. There are two sources for H2S production: the reaction of removing hydrogen sulfide and deamination after proteolysis, and the deoxidizing of SO4- from sulfates in the substrate. It is essential to note that H2S can become sulfuric acid when it dissolves in water. Sulfuric acid is highly corrosive and can severally damage CHP equipment. H2S is also toxic and as such poses personnel safety concerns. Although both CH4 and CO2 are gases without color, odor and toxicity, biogas has odor and toxicity largely due the presence of H2S.
The current methods of biogas desulphurization include chemical, chemical-biological, biological and physiochemical processes. For most biogas-to-power applications, the H2S yield from the digester is under 5,000 ppm. At this level, a bio-desulphurization approach should be considered. The only exception is for applications involving the fermentation of molasses spent wash, where the H2S yield could be as high as 30,000 ppm. For such applications, a chemical-biological system is recommended.
Bio-desulphurization (as shown in figure above) refers to the process that converts H2S into S through the metabolizing effect of desulphurization bacteria under appropriate temperature, humidity and slight oxygenation. The reaction process is:
H2S + 2O2 → H2SO4
2H2S + O2 → 2S + 2H2O
The key point of the bio-desulphurization method is controlling the concentration of dissolved oxygen in the reaction device based on the changes of the H2S concentration and the redox potential.
The main advantages of using biogas desulphurization compared to chemical and physical methods are:
- Higher efficiency
- Lower operational cost
- Easier operation with minimal secondary pollution
Gas Storage
In medium to large biogas projects, the output of biogas is sometimes variable due to fluctuating feedstock processing rates. To reasonably and effectively balance biogas production and consumption, gas storage is often adopted.
The volume of the gas storage tank used for power projects is typically calculated based on 10% of daily gas production.
A double-membrane, dry-type biogas storage tank (figure above) is mainly made up of an outer membrane, an inner membrane, a basal membrane and a concrete foundation. The cavity between the inner membrane and the basal membrane is used for biogas storage. The space between the outer membrane and the inner membrane is airtight. The outer membrane is inflated to a shape similar to a sphere. The gas storage tank is also equipped with an explosion-rated blower that can auto-regulate the gas input/output to keep steady air pressure on the gas tank.
The inner and outer membranes and basal membrane are formed by using an HF fusion process. The materials have special surface treatment with addition of high-strength polyester fiber and acrylate varnish. This type of gas storage tank has many advantages: it can resist ultraviolet rays and prevent leakage, the membrane is resistant to corrosion and to reaction with the biogas, and the tensile strength is high. Moreover, double-membrane gas storage tanks are safer than water-sealed gas storage tanks, covered lagoons and other forms of storage.
In summary, a functional, reliable, operable biogas system must be well designed. Every stage of the biogas system, including pretreatment, fermentation, nutrient dosing, separation, desulfurization and storage, must be properly designed and maintained.